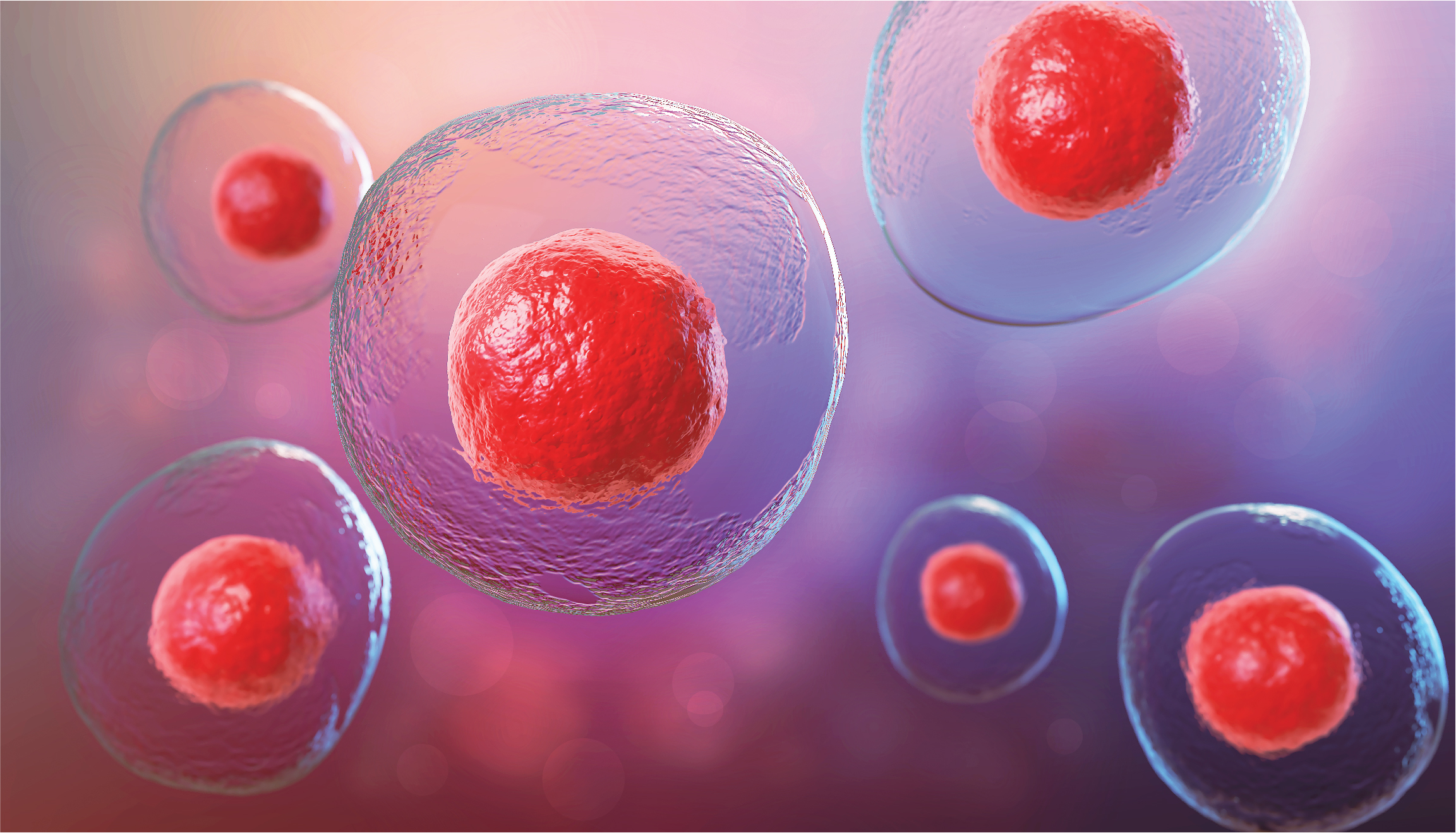
PE IVIS XRMS System for multi-species optical and X-ray Imaging was used to perform live imaging of tumours developed in Raji-Luc Disseminated model implanted with metastatic A549-LuC cells. The Luciferase signals captured intensified over 6-18 days of tumour implantation, whereas the signals were weak in treated mice. Similarly, luciferase signal was captured in lungs in intraperitoneal or sub-cutaneous tumours in SKOV-3 xenograft model and IntegriSenseTM 645 signal in BX PC3-Luc orthotopic model.
Live Imaging of Raji-Luc Disseminated Model
Live Imaging of A549-Luc Lung Metastasis Model
Living Imaging of BX PC3-luc Orthotopic Model
Live Imaging of SKOV-3 xenograft with IntegriSenseTM645
This study in murine tumor shows the early detection of SK-OV-3 Luc ovarian cancer cells implanted sub-cutaneous regions before they form clearly visible tumors. Such a study might give a tumor development timeline that may be used to develop a window for treatment with anti-cancer therapeutics. The graphs show the increase in tumor volume over time and the treatment with Trastuzumab reduced the tumor growth. Luciferase imaging intensity was high in untreated mice while the intensity decreased in treated mice, suggesting the anti-tumor effect of Trastuzumab. Also shown is the workflow for this study developed in consultation with the clients or partners.
IntegriSense is a targeted fluorescent imaging agent comprising a potent, selective non-peptide small molecule integrin avb3 antagonist tagged with NIR fluorochrome. It was developed to enable in vivo visualization and quantification ofintegrin expressed in tumor cells as well as in neo vasculature, and monitor tumour growth, tumor angiogenesis and treatment efficacy.
This study in murine xenograft model shows the images of sub-cutaneous SK-OV-3 tumor at 1-48 hrs. post IntegriSense TM 645 administration (100 mL/mouse) via tail vein to 2 mice (LEFT) and vehicle administered to 1 mouse (RIGHT). Note that the tumor is undetectable in pre-IntegriSense TM 645 administered mice
We performed Immunophenotyping in different call lines using Attune (14 colour FACS) as shown in the following table. Different subsets of immune cells were tagged, and different T cell counts were measured.
Methodology: Immunophenotyping couples’ specific antibody to a fluorescent compound and helps in measuring specific protein expression within a cell population. The protein expressed in certain cells are used to identify and categorize the population of tagged cells. It is often used to measure CD4-T cell counts in specific immunodeficiency disorders and immune-related diseases. It is also used to detect the presence or absence of biomarkers of cancer in cancer cells to predict their severity in forming tumors and to study targeted drug effects.